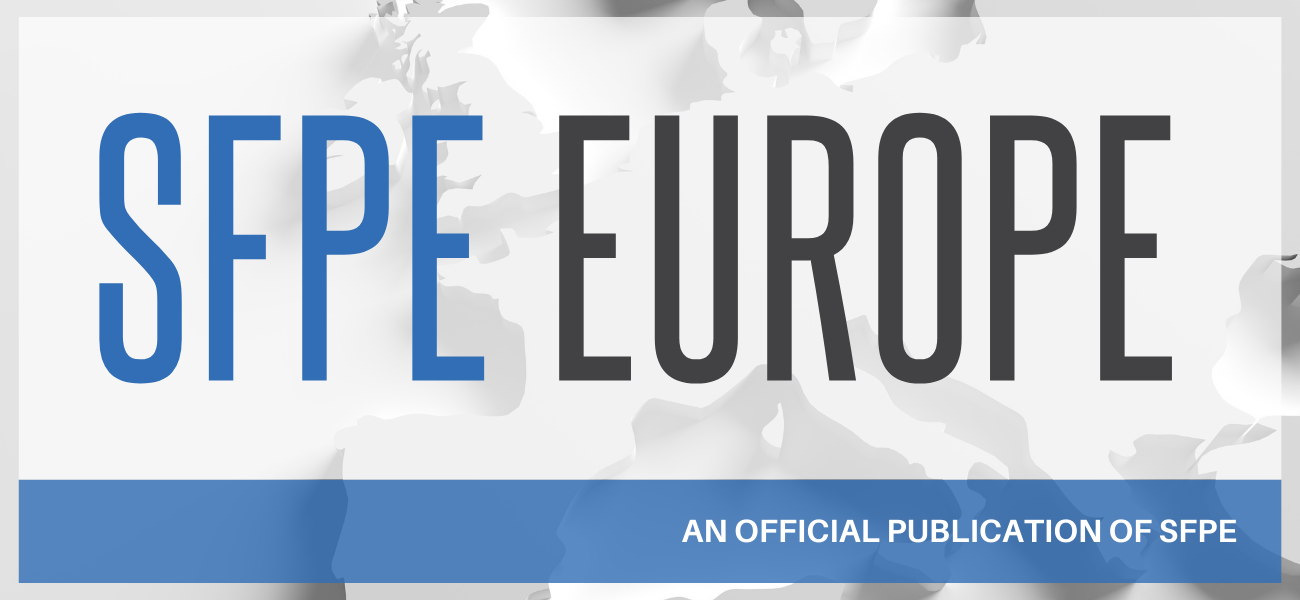
View the PDF here
Enhancing Fire Dynamics Education and Research: A New Tunnel Fire Research Facility
Lund University has funded the construction of a tunnel facility for fire research in Revinge about 20 km east of Lund, Sweden. The facility is located on premises of the Swedish Civil Contingency Agency college where education and training in the field of societal safety and preparedness takes place. However, the tunnel facility is owned and managed by the Division of Fire Safety Engineering at Lund University.
The main structure consists of a 60-meter-long tunnel/corridor with a room connected. The entire structure is made from repurposed shipping containers (see Figure 1 and Figure 2), which have an interior that is partly lined with lightweight concrete. There is also a separate structure for hydrogen safety research on the same site. Although tunnel fire dynamics constitute a significant area of research, the facility presents numerous opportunities for both fire science research and education.
Figure 1. The 60 m long corridor with a connected room constructed with shipping containers
Figure 2. Cross-section of the tunnel (left) and a thermocouple tree in the tunnel (right). Pictures by Larsson and Gondinger [1].
Educational purposes
The fire engineering education at Lund University has experienced substantial growth and improvement in recent years, exemplified by the revision of existing courses and the introduction of new ones. Furthermore, the establishment of the new fire research facility provides students with access to state-of-the-art fire laboratories.
As an example, the new tunnel facility is used in one of the labs in the fire dynamics course for the second-year students. In the development of the laboratory an important aspect was to create relevant scenarios. In contrast to the other laboratory in the course where the students study fire dynamics in a 1:4-scale ISO room, the tunnel fire laboratory offers a more targeted and relevant scenario in terms of fire engineering for aspiring fire safety engineers. By focusing on scenarios that mirror potential real-world situations, the new lab aligns closely with the principles of performance-based fire engineering.
Two scenarios are studied in the laboratory: the first, a room fire scenario where smoke propagates into the corridor (see Figure 3), and second, a corridor fire scenario leading to smoke spread into the room (see Figure 4). The ventilation conditions can be changed and adapted in the room and corridor by opening and closing purposely built hatches and doors.
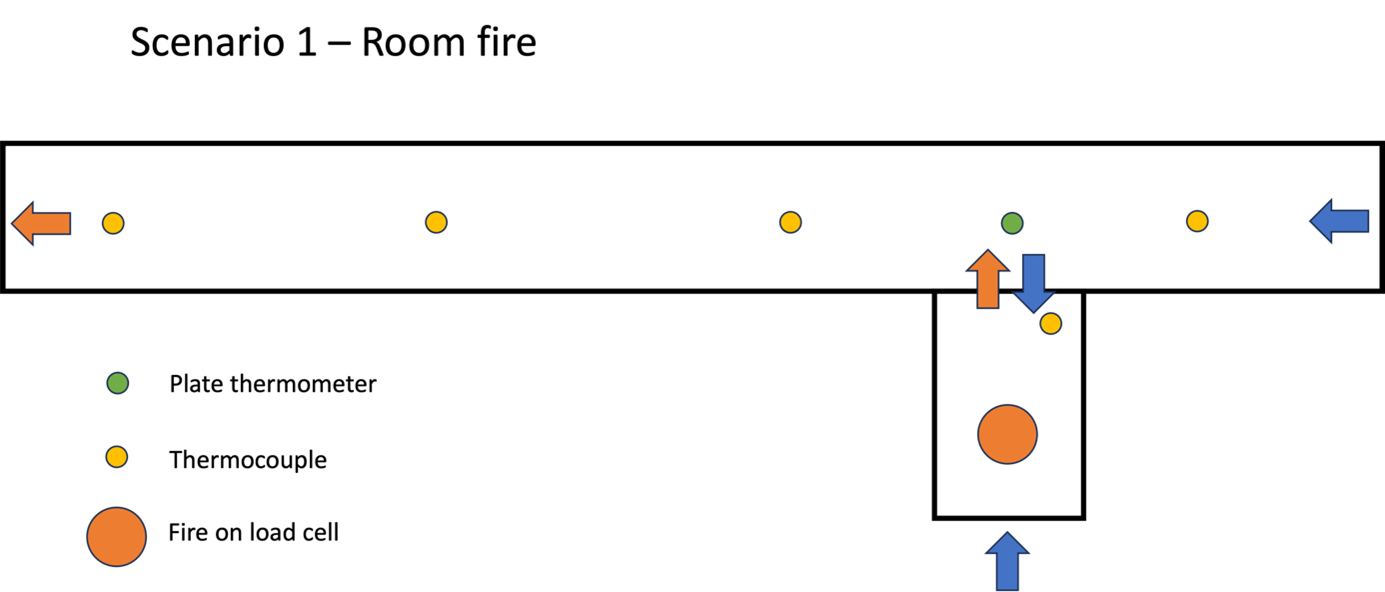
Figure 3: First scenario in the student laboratory.
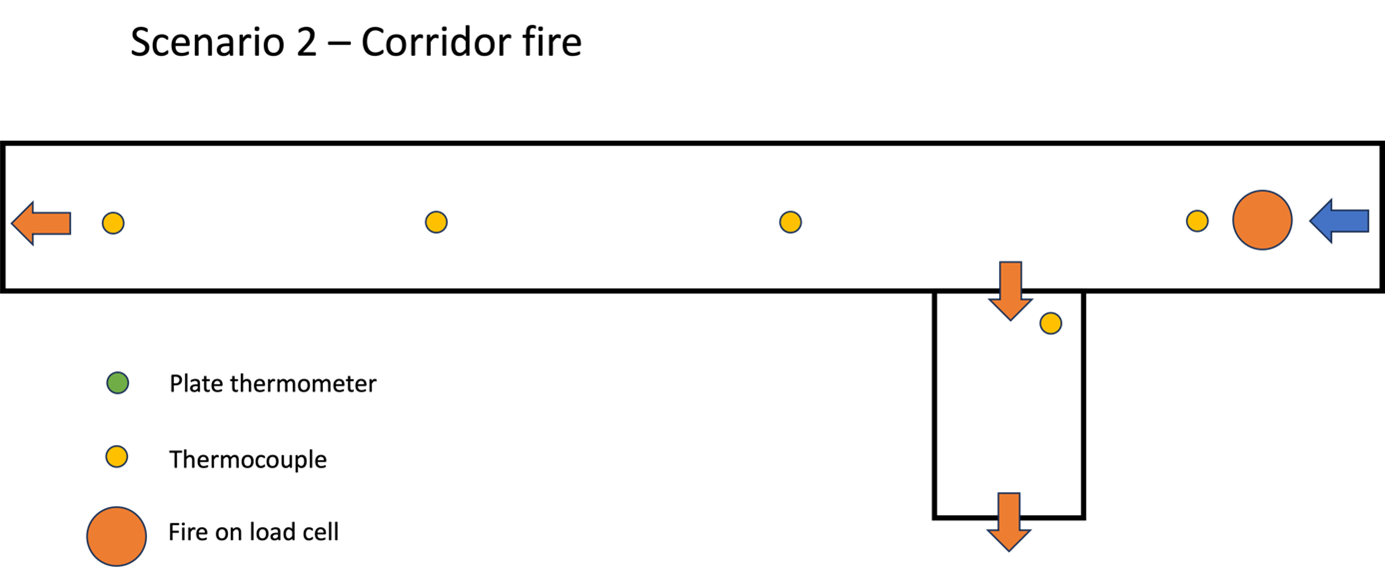
Figure 4: Second scenario in the student laboratory.
Through hands-on experimentation, students gain valuable experience in observing and analysing the complexities of fire dynamics, enhancing their understanding of fire behaviour and the efficacy of different fire models (applicable hand-calculations, zone-models and CFD models) in predicting real-world outcomes. This laboratory exercise fosters critical thinking regarding the use of models and problem-solving skills essential for future courses in fire safety engineering and related areas.
Further, the facility is anticipated to serve as a resource for laboratories in other courses within the local fire safety engineering program at Lund University. However, these laboratories and courses are still in the developmental stages.
Research purposes
The facility presents promising opportunities for different research studies. In an initial research study [1] the repeatability of a large-scale tunnel fire scenario was investigated. Moreover, the effectiveness of different computational methods in predicting tunnel fire conditions were assessed based on the data from the experimental tests, aiming to discern the methods respective strengths and limitations.
In the experimental tests the facility was equipped with thermocouples (five trees with seven thermocouples in each) and plate thermometers positioned to monitor and record temperature at various points during tests (see Figure 4). Additionally, to accurately measure the mass loss rate the fire source is placed atop a load cell, providing data of the fuel consumption over time. A positive pressure fan was placed in on one side of the tunnel to simulate a longitudinal air flow in the tunnel. The fan generated a flow of 2 m/s in the tunnel. The fire consisted of around 20 litres of heptane in a pan with a diameter of 570 mm. The heat release rate was estimated to be 600-700 kW during the tests.
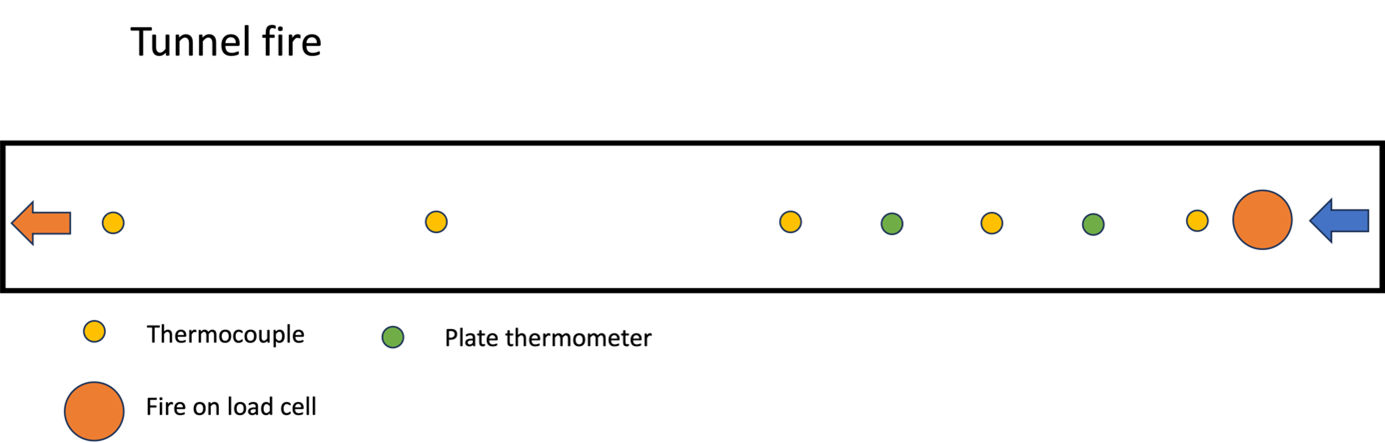
Figure 4: Principal sketch of setup during one possible setup for a tunnel fire test series.
The comprehensive results and analysis of both the fire tests and the corresponding modeling efforts are presented within a master's thesis [1], to which the reader is directed. Nonetheless, for immediate reference, some relevant test data is provided in the following graphs (Figure 5 and 6). In Figure 6 an average temperature during 400-500 seconds into the tests have been used, since reasonably stable temperature conditions are estimated to have been established in the tunnel during this period.
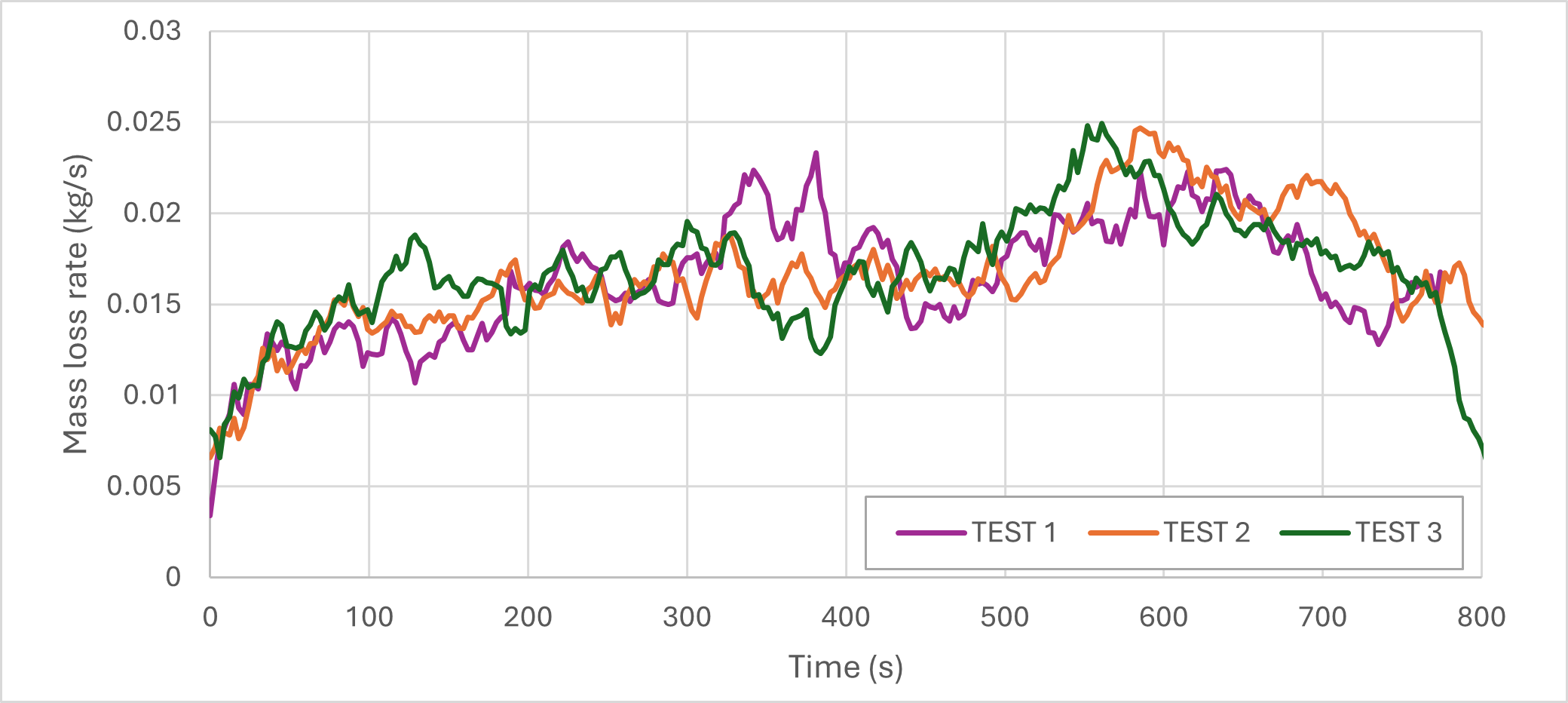
Figure 5: Mass loss rate in the three conducted initial tunnel fire test series.
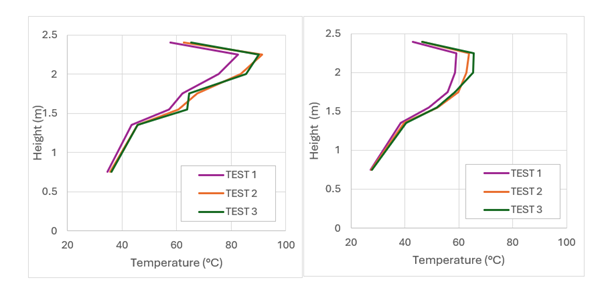
Figure 6: Vertical temperature profile at 18 m (left) and 42 m (right) from the fire source.
Regarding the repeatability of the conducted large-scale experiment, it was generally good. For the mass burning rate, repeatability was good, with similar results obtained from the three experiments showing only minor differences (see Figure 5). Concerning temperature, the results were similar for tests 2 and 3, while test 1 had larger deviations (see Figure 6). The temperatures in test 1 were lower than in the other two tests, possibly because, the tunnel was used for the first time in test 1 and moisture in the inner lining could have been present, consuming energy for moisture evaporation.
In a real-world case, there may be moisture in the tunnel walls. In this regard, test 1 may be the test with the best external validity. However, in comparing the results with simulations, tests 2-3 could be considered to be more relevant since the results correlate well. Even so, accurately predicting the thermal properties of materials under tunnel fire conditions is a significant challenge. The presence of moisture in the tunnel walls, introduces another layer of complexity. This moisture can affect the thermal response and structural integrity of the materials in ways that are difficult to predict. Even with sophisticated simulation techniques, it can be hard to accurately model tunnel fire tests. This difficulty underscores the importance of ongoing research, and the performed fire tests will be reproduced in order to get a better understanding of the possible variability and experimental uncertainty in this type of fire test.
Conclusion
By transitioning to this updated laboratory setup in fire engineering education, students are better equipped to apply theoretical knowledge to practical scenarios, preparing them for the complexities of modern fire safety engineering practices.
Preliminary findings from the tunnel facility testing reveal a generally high level of repeatability, particularly evident in well-defined fire scenarios such as those involving heptane. This reliability is crucial for accurately assessing experimental uncertainties and establishing a robust dataset for future model validation purposes.
References
[1] Larsson, A. & Gondinger, J. Case study on tunnel fires - An experimental study of the fire progression in a tunnel: consistency between simulation, calculation, and experiments. Division of fire safety engineering, Lund University, Sweden.