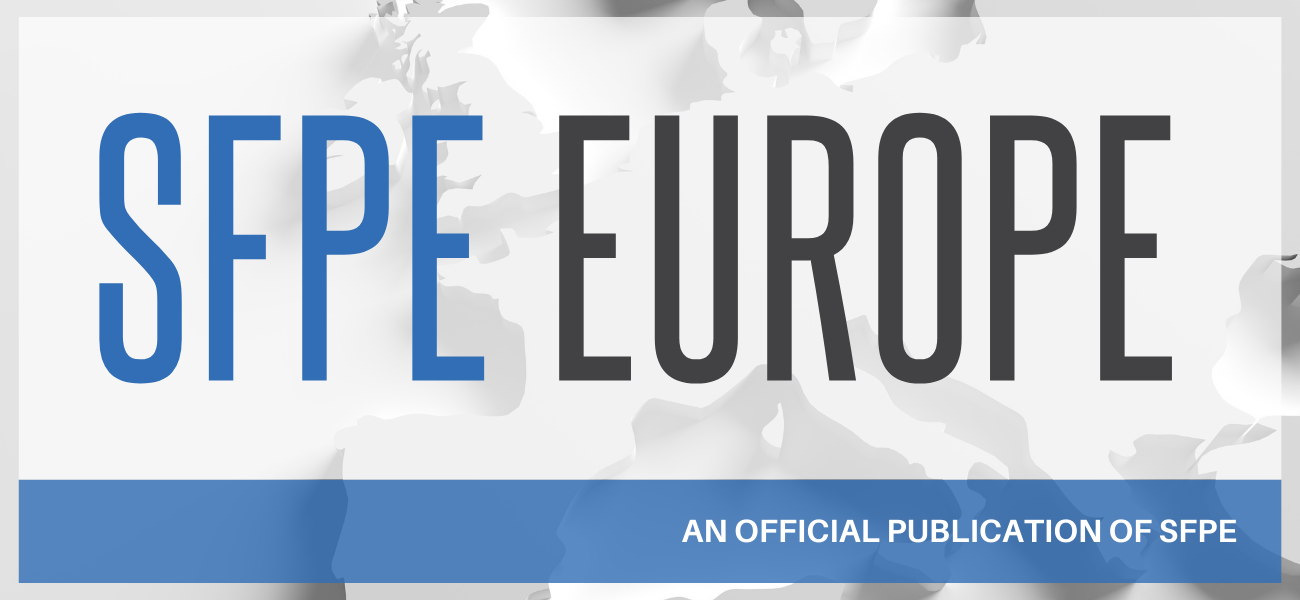
View the PDF here
Landscape of Battery Energy Storage System Hazards and Mitigation Strategies
By: Victoria Hutchison, Fire Protection Research Foundation
The world is undergoing a dramatic energy transition. In response to the pledged action against climate change, countries are aiming to attain net-zero emissions by 2050 [1]. Given that traditional energy production mechanisms contribute to over 75% of the greenhouse gas emissions and 90% of CO2 emissions, investment in clean, alternative sources of energy has become vital [2]. With increasing rolling brownouts, prolonged blackouts and aging electrical infrastructure – lithium-ion battery energy storage systems are proliferating at an exponential pace and are serving a critical role in making the grid more resilient.
While energy storage has become an essential building block of the modern electrical grid, serious safety concerns have emerged as a result of a handful of high-profile fires at large lithium-ion battery energy storage facilities. Thus, a comprehensive approach to BESS fire safety is required to fully unlock the potential of these systems in the energy transition.
To support the fire protection engineering community, the Fire Protection Research Foundation initiated a project supported by the members of the FPRF Energy Storage Research Consortium (ESRC), led by Jensen Hughes, to define the landscape of lithium-ion battery-based energy storage system deployments, their hazards, consequences, and the factors that should be considered for a comprehensive protection and hazard mitigation strategy.
ESRC members: Energy Safety Response Group (ESRG), Electric Power Research Institute (EPRI), Fire & Risk Alliance, LLC, FM Global, General Motors, Pacific Northwest National Labs (PNNL), Southern Company, Tesla, UL Research Institutes, and Wartsila Energy.
Landscape of BESS Systems
As part of this study, the Fire Protection Research Foundation (FPRF) conducted an international survey in 2022 to get insight into the landscape of commercial li-ion battery ESS applications. The energy storage systems characterized through this study are generally summarized into one of the following categories:
· ESS walk-in unit (or container with accessibility)
· ESS cabinet (or container without accessibility)
· ESS dedicated-use building
The majority of the surveyed ESS installations were container-based (with and without access). Of the 140 responses, 66% were located outdoors, 49% were located in rural areas and 65% placed more than 30 m (100 ft) from exposures. The total power capacity of the surveyed sites ranged from less than 0.3 MW to over 125 MW. The lithium-ion battery cell chemistries identified through the survey were NMC (Lithium Nickel Manganese Cobalt Oxide – 36%), LFP (Lithium Iron Phosphate – 29%), LCO (Lithium Cobalt Oxide – 12%), NCA (Lithium Nickel Cobalt Aluminum Oxide – 9%), LMO (Lithium Manganese Oxide – 8%), and others (6%).
In short, the landscape of Li-ion BESS systems is broad.
Hazards
Lithium-ion BESS hazards can generally be categorized as fire and explosion hazards, chemical hazards, electrical hazards, stranded energy hazards, and physical hazards. This study, however, focused on fire and explosion hazards, specifically, the phenomena of thermal runaway, due to it being the hazardous condition with the greatest potential for severe consequences. Thermal runaway is one of the dominant risks associated with lithium-ion batteries and is defined as an exothermic reaction in a lithium-ion cell that enters into an uncontrollable self-heating state that results in extremely high temperatures, violent venting of flammable and toxic gases, shrapnel and particulates, smoke and fire. Once initiated in one cell, thermal runaway propagation may ensue and spread to adjacent cells, modules, and potentially beyond depending on the level of protection in place and its effectiveness in limiting spread. If thermal runaway is allowed to propagate, an extensive amount of flammable gas can accumulate and pose an explosion or fire hazard.
Mitigation Strategies
Mitigation strategies are typically put in place to help prevent batteries from entering thermal runaway, manage the byproducts or effects of thermal runaway, and provide cooling to slow the cascading effects to other cells within a module or rack. The unique challenge for protecting a li-ion battery ESS is that it presents both a fire and explosion hazard simultaneously. The designs for most fire suppression strategies, like sprinkler systems, assume ignition. If the BESS fails to ignite, the off-gassing due to thermal runaway can then present an explosion hazard. Thus, traditional mitigation strategies can be challenged when protecting BESS due to constantly evolving technology and designs, unique hazards of thermal runaway, extended duration events, poorly defined protection goals, and limited proven mitigation strategies. A one size fits all prescriptive solution does not exist for BESS, thus requiring designers and engineers to think differently. Rather than simply following prescriptive guidance, facility owners/operators, engineers, designers, or other applicable stakeholders must consider, “What is the risk tolerance or acceptable loss this facility? One module? One rack? Entire container or building?”
Strategies for BESS hazard mitigation should be considered through the establishment of protection goals and developing comprehensive mitigation strategies around prevention, managing the impact, and/or managing the exposed. Commonly used mitigation strategies to support these respective goals are identified in Table 1.
Table 1: Types of ESS hazard mitigation strategies for respective goals of prevention, managing the impact or managing the exposed.
Prevent the Incident
|
Manage the Impact of Fire/Explosion
|
Manage the Exposed
|
- Battery Management System
- Energy Management System
- Power System Protection
- Early Detection
- Safety through Design
- Fire Prevention Practices
|
- Detection Systems (smoke, heat, air sampling, gas, and infrared flame detection)
- Automatic Protection Systems (e.g., automatic sprinkler system, water mist, clean agent, CO2, aerosol)
- Manual Suppression (e.g., dry pipe deluge)
- Explosion Protection (mechanical exhaust, deflagration venting)
- Fire Department Response
|
- Manual Suppression
- Implement Emergency Response Plan
- Fire Department Response
|
All mitigation strategies for battery energy storage systems have their own advantages and limitations that should be considered in the design process. An analysis of several of the active mitigation strategies deployed in BESS installations, and their role in hazard mitigation, are summarized in Table 2.
Table 2: Types of active ESS hazard mitigation strategies with corresponding advantages and limitations
Category
|
Name
|
Advantages
|
Limitations
|
Explosion Prevention/ Protection
|
Mechanical Exhaust
|
- When activated with gas detection signal, venting the enclosure may prevent formation of explosive concentrations.
|
- Will not prevent or stop thermal runaway conditions.
|
Deflagration Venting
|
- Passive protection to prevent severe explosion/deflagration.
|
- Failure of the container content may not be prevented. Mostly for isolating damage to one BESS (i.e., container) without a severe explosion.
|
Fire Suppression*
|
Automatic Sprinkler Systems
|
- Fire control and suppression for flaming fires.
- Provides thermal management capability (i.e., cooling of the environment)
|
- Not expected to stop thermal runaway propagation as water may not reach the cells. Cell to cell or module to module thermal runaway propagation may not be stopped.
- Water runoff concerns may need to be addressed as part of the design or during the event to meet local regulations.
|
Water Mist
|
- Fire control and suppression for flaming fires.
- Provides thermal management capability (i.e., cooling of the environment)
|
- Not expected to stop thermal runaway conditions.
- Depending on the system design, water runoff concerns may need to be addressed as part of the design or during the event to meet local regulations.
|
Clean Agents
|
|
|
Aerosol or inerting System
|
- Fire control and suppression for flaming fires.
|
- Not expected to stop thermal runaway conditions.
- May not suppress the fire as the agent concentration may not hold or be maintained given the off gassing from the batteries.
- Does not provide thermal management (i.e., cooling of the environment)
|
Manual Spray System
|
- Fire control and suppression for flaming fires. Control of fire propagation.
|
- Application expected to be late in the event. Mostly intended to prevent propagation to nearby BESS, cooling of the BESS on fire and cooling adjacent BESS.
|
Fire Brigade/Fire Department Intervention
|
- Fire control and suppression for flaming fires. Control of fire propagation.
|
- Application expected to be late in the event. Mostly intended to prevent propagation to nearby BESS.
- Introduces first responders to hazards when the equipment is likely destroyed and, in many cases, there is no life safety at risk.
|
Fire Detection
|
Smoke detection
|
- Detection of flaming fires in early stages.
|
- Not expected to prevent or detect thermal runaway conditions.
- Not expected to detect the onset and early stages of thermal runaway.
|
Heat detection
|
- Detection of flaming fires in early stages. Can be used for activation of automatic suppression.
|
- Not expected to prevent thermal runaway conditions.
- Not expected to detect the onset and early stages of thermal runaway.
|
Air sampling
|
- Can detect very early stages of a potential fire and allow time for system shutdown and inspection before thermal runaway progresses.
|
- Not expected to prevent thermal runaway conditions.
- Not expected to detect the onset and early stages of thermal runaway unless specifically configured for detecting such exhaust products.
|
Gas detection
|
- Can detect early stages of thermal runaway conditions and provide signals for system shutdown and the start of automatic ventilation (if available).
|
- Not expected to prevent thermal runaway conditions.
- Depending on system configuration, may be prone to false alarms. Not reliable indication in harsh conditions to monitor concentrations inside a container (e.g., later in an event when detectors may be exposed to severe fire conditions)
|
Infrared/Flame detection
|
- Can detect the onset of flaming fires.
|
- Not expected to prevent thermal runaway conditions.
- Not expected to detect the onset and early stages of thermal runaway.
- May be prone to false alarms.
|
* Suppression systems may be in place within a BESS for purposes other than management of thermal runaway conditions. The limitations stated in this table are only applicable to thermal runaway scenarios.
Other mitigation strategies, beyond active systems, also play an important role in minimizing the consequences of a BESS incident, including BESS management systems intended for incident prevention and the development of emergency response plans pre-incident to ensure the fire department is prepared for efficient response.
Insights from the survey conducted as part of this study, found about half of the surveyed BESS containers to have fire detection, active protection (e.g., automatic sprinklers, clean agent, etc.), and/or explosion mitigation (i.e., deflagration venting or explosion prevention). For other BESS unit types, active protection systems appeared to be less common.
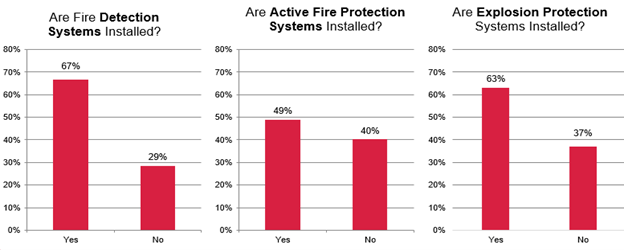
Figure 1: Survey Results for installed mitigation strategies.
For outdoor installations, 48% had both suppression and detection systems installed, 34% had neither suppression or detection systems installed, and 7% only had detection. It should be noted that 77% of those without any suppression or detection systems were in BESS containers without accessibility. Indoor systems surveyed appeared to have a slightly higher presence of active mitigation strategies, with 52% having both suppression and detection, 23% having neither suppression or detection and 19% having only detection. Out of the 63% of facilities having passive protection installed, half had deflagration venting whereas the other half had explosion prevention systems installed.
The most common detection strategy appears to be a combination of detectors (47%), with some combination of gas detection, heat/smoke detection, flame detection and air sampling. For active protection or suppression systems, 40% of surveyed installations utilized a water-based suppression agent, with 45% of those installations utilizing a local application water-based solution. 35% used a clean agent system, of which 36% were combined with sprinklers. Additionally, 12% utilized an aerosol-based agent, 75% of which were combined with another backup suppression system, typically a dry pipe or manually activated sprinkler system. When selecting an optimal suppressant for BESS installations, it is imperative for the evaluation to focus on the heat mitigation potential at the module/unit level, protection of BESS from external fires, gas absorption capabilities, and gas temperature reduction capabilities.
Taking the advantages and limitations of the available mitigation strategies into consideration, a comprehensive protection strategy, based on the established protection goals and acceptable level of risk, is recommended to include the following:
- A hazard mitigation analysis (HMA) to inform applicable stakeholders of the hazards and applicable mitigation strategies associated with the BESS installation.
- An emergency response plan (ERP) including training for first responders and environmental impact management.
- A BMS, or similar protection system for continuous monitoring of the battery and overall system condition with the ability to shut down the system if deviation from normal operations is detected.
- A gas detection system to trigger the operation of explosion prevention or control measures.
- A fire detection system (e.g., heat, smoke or both).
- A fire suppression system, with the ability to flood the BESS.
- Deflagration venting protection.
- Emergency response by fire department.
Specification of appropriate spacing between BESS, access for emergency response and requirements for managing runoff fire water should also be considered as part of the comprehensive protection strategy.
Conceptual And IDEAL Characterization of Li-ion BESS Events
Through review of BESS failure modes, hazards, available mitigation strategies and incident case studies, a generic framework that characterizes a BESS incident was developed to serve as the basis for informing BESS mitigation strategy design, from the prevention of failure modes that may trigger thermal runaway to the mitigation of consequences once thermal runaway starts.
The resulting consequences of BESS incidents can be characterized by a set of common elements and their interactions between ignition sources, other combustibles, fire protection features, human factors, facility configuration and other elements. These elements can be conceptually represented as a sequence of events over time to serve as a guide for understanding the extent of damage (i.e., the consequences level) in a given scenario, based on the success or failure of mitigation strategies in comparison to an incident’s consequence without intervention. Therefore, to explicitly account for the impact of various mitigation strategies on a BESS incident, a number of possible outcomes with progressively larger consequences can be evaluated by superimposing the effects of mitigation strategies over an incident timeline (e.g., Fig 2).
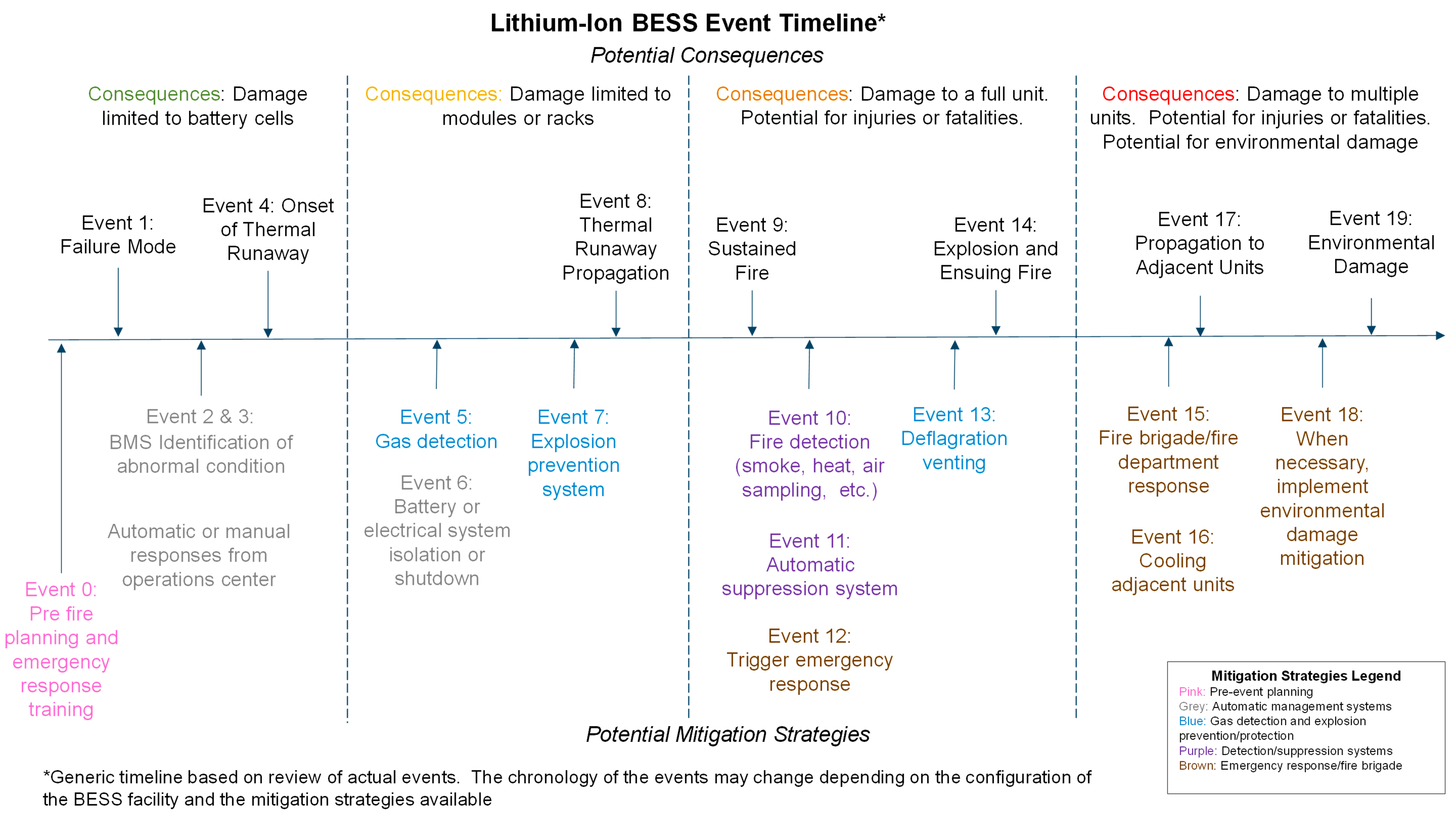
Figure 2: Li-ion BESS Event Timeline
Figure 2 is a representative sample of a BESS event timeline and consequences, informed by review of past incidents, however, this could change based on the specific scenarios, sequence of events, protection strategies in place, etc. Consequences can vary widely, ranging from the onset of thermal runaway that is quickly controlled producing only localized damage to cells to extensive flaming, deflagration or explosion conditions. Therefore, the basis for design and implementation of BESS protection strategies can be informed by assessments of scenario progressions, with consideration of the impact of mitigation strategies on the potential consequences and the alignment with protection goals.
Knowledge gaps
While knowledge of the hazards of these systems continues to increase, an extensive number of unknowns still exist, including a lack of recommended best practices. This study documented fifteen areas of future research that can serve to close knowledge gaps in the state-of-the-art understanding of safe BESS operations. Management of potential environmental impacts, both airborne and water runoff, efficiency of active protection systems, protection for residential energy storage system applications, and recommended response strategies and training for emergency responders emerged as the highest priority research gaps.
In summary, research study suggested that the landscape of li-ion battery energy storage system developments are broad and best practices for mitigation strategies are still lacking. However, this study provides baseline information that assists in the decision-making process for developing a comprehensive protection strategy for BESS facilities.
References
[1] United Nations (2023). Climate Action. Retrieved from: https://www.un.org/en/climatechange/net-zero-coalition
[2] European Commission. (2021). Questions and Answers - Making our energy system fit for our climate targets. Brussels. Retrieved from: https://ec.europa.eu/commission/presscorner/detail/en/qanda_21_3544
[3] Joglar, F. (2023). Landscape of Battery Energy Storage System Hazards and Mitigation Strategies. Fire Protection Research Foundation, Quincy. (to be published)
[4] NFPA (2023). NFPA 855, Standard for the Installation of Stationary Energy Storage Systems. Quincy.